Bioremediation of Manufactured Gas Plant Sites
This document contains information about the bioremediation of contaminated soils and is intended to help teachers improve their content knowledge of bioremediation. Background information addresses the question of whose responsibility it is to clean up pollution. The story of how soil can come to be polluted is explained by the history of manufactured gas plant (MGP) sites. MGP sites are industrial sites which were active during the 19th and early 20th centuries. The final part of this document discusses the research of the featured scientist, Dr. Sabrina Powell. She investigated a technique to improve the cleanup of contaminated soils at MGP sites. The text shows the application of the scientific method to a real-world problem.
Background Information
We can all agree that people need a clean environment—air, water, and soil. A clean environment keeps people healthy. How do you define “clean”? Who is responsible for ensuring that the environment stays clean? We all share responsibility for keeping the environment clean. The government has laws to ensure that everyone treats the earth well. Congress passes environmental laws in areas such as drinking water quality, air quality, and wetland management. The Environmental Protection Agency (EPA) enforces these laws and determine how the laws will be put into practice. For example, sulfur dioxide is an air pollutant identified in the Clean Air Act passed by Congress. The EPA decides what levels of sulfur dioxide are safe, sets limits for how much sulfur dioxide industries can legally emit into the air, and determines penalties for exceeding these emission limits. The EPA works with industries and individuals to make sure the law is obeyed.
At the state level, the Florida Department of Environmental Protection (DEP) is responsible for environmental management and stewardship. The DEP’s primary goal is to protect Florida’s air, water, and land. The DEP’s work includes restoring the Everglades, improving air quality, restoring and protecting water resources, and conserving environmentally-sensitive lands.
The EPA and DEP work to protect both the environment and human health. These agencies have a twofold mission: preventing pollution before it happens and cleaning up pollution that has already occurred. What is pollution? One definition is: The contamination of air, water, or soil by substances that are harmful to living organisms (as defined by The American Heritage Science Dictionary, Houghton Mifflin Company). Pollution can occur naturally, for example through volcanic eruptions, or as the result of human activities, such as the spilling of oil or improper disposal of industrial waste.
How does pollution get cleaned up? Who pays for the cleanup? If the individual or company who caused the pollution at a contaminated site can be identified, then the individual or company is held responsible for cleanup. If no responsible party can be identified, Congress has passed a law providing funding for the cleanup. The common name for this law is Superfund, while the official name is the Comprehensive Environmental Response, Compensation, and Liability Act. The purpose of the Superfund Act is to protect people and communities from heavily contaminated toxic waste sites that have been abandoned. The money in the Superfund comes from a tax on chemical and petroleum industries. There are currently about 1200 sites on the Superfund list, and about 300 sites have been cleaned up since 1980. Ironically, despite its name, the Superfund lacks the funds to clean up even a small number of sites on the Superfund list. The Superfund list serves primarily informational purposes, identifying for the public those sites which need to be cleaned up.
The History of Manufactured Gas Plant Sites
What is manufactured gas? Prior to the advent of electricity, manufactured gas served as a fuel source for cooking, heating, and lighting. Manufactured gas is similar to the propane that fuels a BBQ grill or a gas stove. Manufactured gas plants (MGPs), facilities where manufactured gas was produced, operated from the early 1800s until the mid 1900s. Typical MGP sites ranged between 1 and 200 acres in size. There are an estimated 50,000 of these sites in the US.
How is manufactured gas made? In the MGP process, coal is combusted (burned) inside a closed chamber. This combustion process generates energy-rich gases. The manufactured gas was captured, stored on site in large tanks, and then distributed to customers via a network of pipes. The typical composition of manufactured gas created by this process is hydrogen (50%), methane (35%), carbon monoxide (10%), and ethylene (5%). This process can be compared to an internal combustion engine in a car, where gasoline is burned.
The production of manufactured gas generated coal tar waste. Coal tar includes tars, oils, benzene, toluene, ethylbenzene, xylene, volatile and semi-volatile organic compounds, phenolics, polycyclic aromatic hydrocarbons, cyanides, thiocyanates, metals (arsenic, chromium, copper, lead, nickel, zinc), ammonia, nitrates, ash, lime wastes, and sulfates/sulfides. Often the coal tar was dumped or buried onsite. Because they are so long-lived, the tar contaminated the soil and groundwater in and around the manufacturing plant. Many MGP sites are a serious environmental concern. Of the estimated 50,000 MGP sites in the US, 60-90% of them have not yet been characterized. Estimated cleanup costs range from $1-100 million per site, for a total of $26-128 billion for the entire country.
Polycyclic Aromatic Hydrocarbons
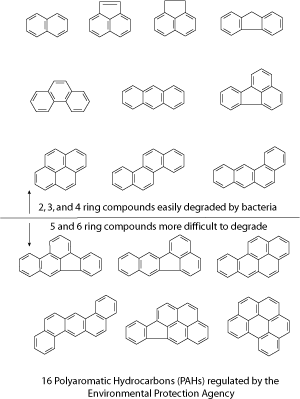
Polycyclic Aromatic Hydrocarbons (PAHs) are one type of contaminants found at MGP sites. Sometimes called polyaromatic hydrocarbons, these compounds are formed of rings, with each ring containing 5 or 6 carbon atoms. Polycyclic is a word that means poly (many) cycles (rings). The chemical bonds that hold the carbon atoms together are formed when electrons are shared between the carbon atoms. The word aromatic refers to a type of bond that is intermediate in strength between a single and a double bond. The word hydrocarbon refers to a compound containing only the elements hydrogen and carbon.
The EPA regulates 16 polyaromatic hydrocarbons as priority pollutants, which means that the release of these chemicals into the environment is not allowed above certain levels. These 16 polyaromatic hydrocarbons range in size from 2 to 6 rings. Because of their low water solubility, polyaromatic hydrocarbons are most commonly found in soil or sediment, rather than in water or air.
In addition to being universally present at MGP sites, polyaromatic hydrocarbons are among the most widespread organic pollutants. During the production of manufactured gas, polyaromatic hydrocarbons are formed from the incomplete combustion of coal. Some polyaromatic hydrocarbons are carcinogens, which means they can cause cancer. A person could be exposed to polyaromatic hydrocarbons through breathing polyaromatic hydrocarbon-containing particles such as tobacco smoke, eating food containing polyaromatic hydrocarbons, or skin exposure. Once a polyaromatic hydrocarbon is inside the body, the liver metabolizes it to compounds which interact with proteins and DNA. The binding of polyaromatic hydrocarbon-compounds to DNA can lead to the growth of cancerous tumors.
Bioremediation of Manufactured Gas Plant Sites
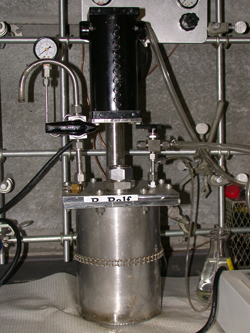
What is bioremediation? A process that uses microorganisms, fungi, green plants, or their enzymes to return the environment altered by contaminates to its original condition. The microorganisms or plants are able to partially or completely degrade the contaminants. Advantages of bioremediation include that it is less expensive than some other methods, it can be done on site, and the contaminants can theoretically be converted to nontoxic carbon dioxide and water. One disadvantage of bioremediation is that the removal of the contaminants is often not complete.
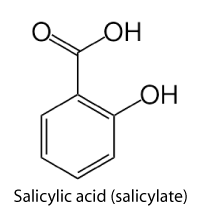
Most polyaromatic hydrocarbons are broken down through microbial degradation rather than abiotic processes. This makes bioremediation a logical choice for cleanup of hydrocarbon-contaminated soil. However, removal of polyaromatic hydrocarbons is often incomplete. As mentioned earlier, the priority pollutant polyaromatic hydrocarbons range in size from 2 to 6 rings. The smaller polyaromatic hydrocarbons with 2-4 rings are commonly degraded by microorganisms, while the larger 5 and 6-ring polyaromatic hydrocarbons are not as extensively degraded. The microorganisms (bacteria or fungi) are able to produce enzymes which break down the polyaromatic hydrocarbons. The microorganisms then use the polyaromatic hydrocarbons as sources of carbon and energy. The polyaromatic hydrocarbons might be only partially degraded, or they might be degraded all the way to carbon dioxide and water. This process is similar to the digestion that occurs when a person eats a banana. Their stomach produces enzymes which digest the banana. The body uses the carbon and the energy in the banana to fuel growth and metabolism. Carbon dioxide is exhaled in the breath.
Bioremediation of soil often occurs in a bioreactor. What is a bioreactor? A bioreactor is any device that supports a biologically active environment. The size of a bioreactor can range from a less than a gallon to hundreds of thousands of gallons. When a scientist or engineer is trying to design a bioreactor for a certain process, they will often start with a small size version to work the bugs out of the process, then a build larger version. This is a picture of a bioreactor named Ralf. Ralf’s job is to bioremediate polyaromatic hydrocarbon-contaminated soil. He sits about 2 feet (60 cm) tall on the lab bench, and he holds about 1/2 gallon (2 liters). How does a bioreactor work? First, polyaromatic hydrocarbon-contaminated soil from a manufactured gas plant site is mixed with water containing nutrients (nitrogen and phosphorus). This mixture is called a slurry. The slurry is poured into the bioreactor, where it is stirred and aerated (this means air is bubbled into the bioreactor). The nutrients and oxygen encourage the growth of microorganisms. Microorganisms (bacteria) naturally occurring in the soil degrade (eat) the polyaromatic hydrocarbons. Ralf is able to remove about 40-50% of polyaromatic hydrocarbons from MGP site soil after 15 weeks. This is a good start, but I wanted to see if this could be improved.
How might I go about this task? I wanted to stimulate the growth of microorganisms that degrade polyaromatic hydrocarbons. After spending time researching what other scientists have tried, I decided to test a compound called salicylate. Salicylate causes some microorganisms to create new hydrocarbon-degrading enzymes. These enzymes can be useful in cleaning up soil contaminated with naphthalene, a 2-ring polyaromatic hydrocarbon. Previous work by other scientists has shown that salicylate can increase naphthalene degradation rates. Perhaps salicylate would increase degradation rates for other polyaromatic hydrocarbons as well.
I spent about three years working on this experiment, trying to answer the question “Does salicylate increase degradation?”. I measured polyaromatic hydrocarbon degradation rates before and after I added salicylate to the bioreactor. I saw that salicylate addition increased naphthalene degradation rates, just as I expected. However, the degradation rates for the other polyaromatic hydrocarbons were not affected by salicylate addition. I had hoped to see increased degradation rates for all the hydrocarbons I tested. Salicylate was not looking promising as a way to improve the bioremediation of this PAH-contaminated soil.
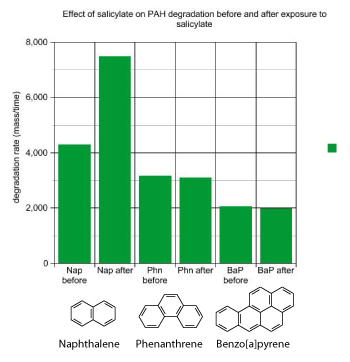
MGP sites are complex, and the cleanup of one of these sites is a complicated process. Often in science, you address a big problem by focusing on a small part of it. Here’s a summary of how I applied the scientific method to polyaromatic hydrocarbons in contaminated soil. My hypothesis was that salicylate addition would increase rates of polyaromatic hydrocarbon degradation in the bioreactor, and this would clean up the soil by removing the polyaromatic hydrocarbons. The results showed that salicylate increased degradation rates for naphthalene, which was consistent with previous research. However, for other polyaromatic hydrocarbons, salicylate addition had no effect on degradation rates. I concluded that the hypothesis was true for naphthalene, but not true for the other PAHs tested. My research with salicylate did not result in an improved method for cleaning up polyaromatic hydrocarbon-contaminated soil, but that’s sometimes how science turns out.
This was disappointing for me, because I had hoped salicylate addition would be useful as a way to improve the cleanup of polyaromatic hydrocarbon-contaminated soils. However, my bioreactor was only using soil from one MGP site, and there’s always a chance that another site, with a different population of microorganisms, could benefit from salicylate addition. Perhaps another scientist somewhere else will try again with another soil and another bioreactor. Perhaps another compound besides salicylate will be found to increase polyaromatic hydrocarbon degradation rates. There are many other scientists working around the world who are researching ways to improve the cleanup of contaminated soil, air, and water. Each scientist contributes a small part to our understanding of the world around us. Science is a community effort, and scientists are always learning from and building on each other’s work.